Research
At Zhu lab, we are pioneering the frontiers of medical innovation through cutting-edge research in light- and radiation-based therapies. Our team is dedicated to advancing Photodynamic Therapy (PDT), leveraging light-activated agents to target and treat cancer with precision, alongside groundbreaking work in Cherenkov Imaging which harnesses Cherenkov radiation to enhance real-time visualization and dosimetry in radiation therapy. We also explore the dosimetry of Photobiomodulation (PBM) to promote tissue repair and reduce inflammation using low-level light. Based at the Perelman School of Medicine, our interdisciplinary approach integrates experimental science, computational modeling, and clinical collaboration to translate discoveries into impactful healthcare solutions. Join us as we illuminate new pathways in precision medicine!
Photodynamic Therapy (PDT)
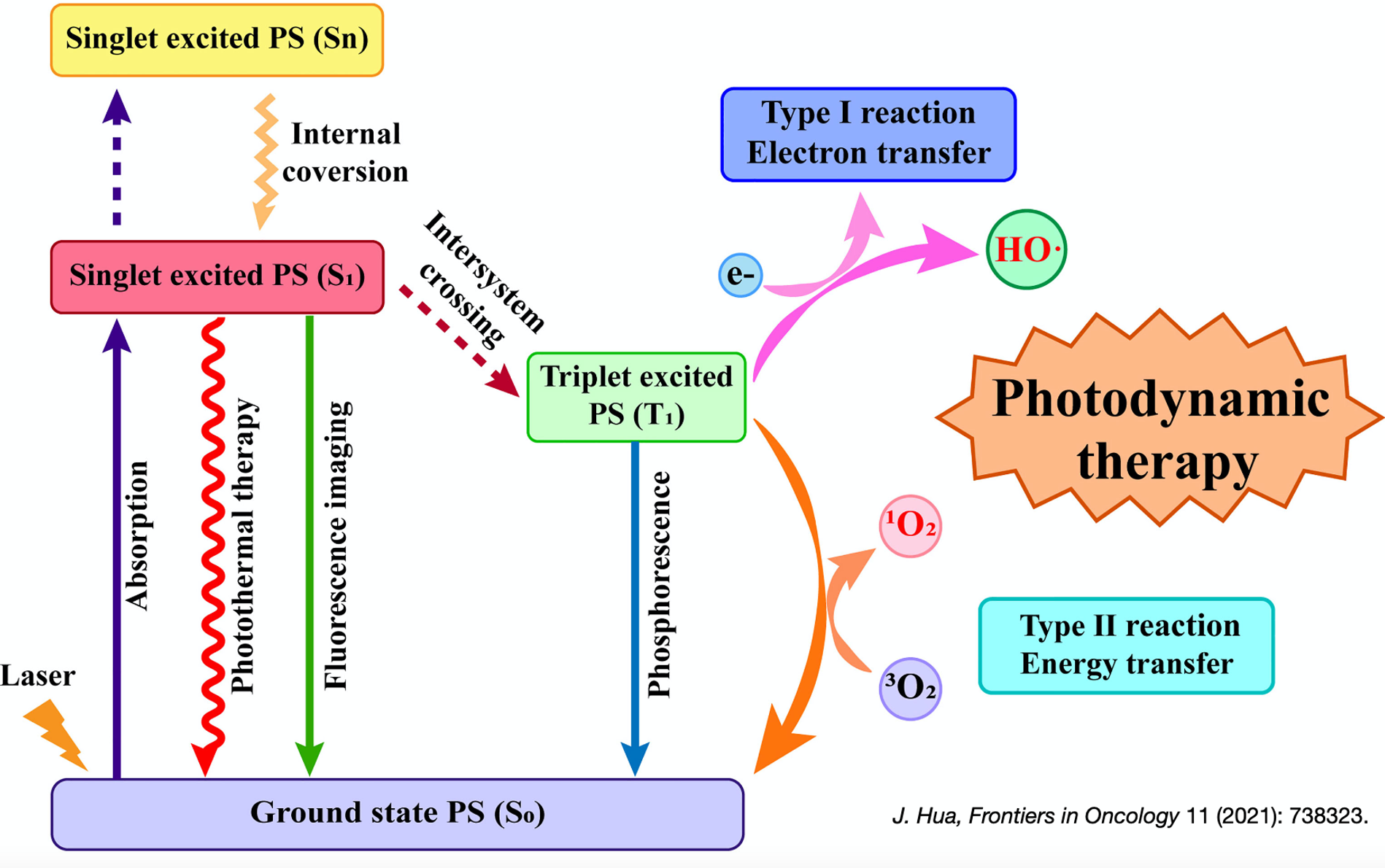
Photodynamic therapy (PDT) is an innovative treatment that combines light-sensitive compounds, known as photosensitizers, with specific wavelengths of light to target and destroy diseased cells, such as in cancer or infections. Our group specializes in the dosimetry of PDT, focusing on optimizing the precise delivery of light and photosensitizer doses to maximize therapeutic effectiveness while minimizing side effects. Through advanced research and techniques, we aim to enhance the accuracy and outcomes of this promising therapy.
Most Recent Research Highlight
Fractionated Photofrin-Mediated Photodynamic Therapy Significantly Improves Long-Term Survival

This study centered on optimizing two-part (fractionated) PDT with a 2 h dark interval by varying the duration of light exposure to cohorts of mice RIF tumors. The primary objective was the 90-day tracking of tumor regrowth as a metric for long-term cure rate. PDT dosimetry includes real-time measurements of tissue oxygenation, photosensitizer uptake, and the light fluence rate to determine the reactive oxygen species concentration [ROS]rx. The study demonstrates that the introduction of a dark interval during treatment substantially increases treatment efficiency, even as other parameters remained relatively consistent.
Correction of Multispectral Singlet Oxygen Luminescent Dosimetry (MSOLD) for Tissue Optical Properties in Photofrin-Mediated Photodynamic Therapy
This study explores the impact of tissue optical properties on singlet oxygen (1O2) luminescence detection in photodynamic therapy (PDT). Using an Avantes spectrometer, we measured 1O2 luminescence in a liquid phantom containing Photofrin, systematically varying scattering and absorption. Singular value decomposition (SVD) effectively isolated the 1O2 signal, and Monte Carlo simulations validated the experimental results. The detected 1O2 signal decreased with absorption and increased with scattering up to 20 cm−1, before saturation. These findings underscore the importance of independently measuring tissue optical properties to enhance PDT dosimetry accuracy.
Cherenkov Imaging
Cherenkov imaging is a cutting-edge technique that visualizes Cherenkov radiation—light emitted when charged particles, such as electrons, travel faster than the speed of light in a medium like tissue during radiation therapy. This non-invasive method allows real-time monitoring of radiation delivery, enabling precise verification of treatment areas and doses. Our group leverages Cherenkov imaging to enhance the accuracy of external beam radiation therapy and total skin electron therapy (TSET), ensuring optimal targeting while minimizing damage to surrounding healthy tissues.
Most Recent Research Highlight
This figure illustrates the detection of Cherenkov signals during radiation therapy using a specialized intensified camera system. The left side of the figure features a DoseOptics C-Dose camera designed for capturing Cherenkov emissions generated by tissue during radiation treatment. Below the camera, a timing diagram shows how the system synchronizes image acquisition with the linear accelerator (Linac) pulses, capturing Cherenkov frames during beam delivery and background frames in between.
On the right side, a series of images illustrate Cherenkov signal detection and its integration with treatment planning. The top left image captures real-time Cherenkov light emissions from a patient’s irradiated breast tissue, while the top right image displays the cumulative Cherenkov signal over the treatment area. The bottom left image shows the planned treatment area, and the bottom right image presents the planned cumulative dose overlaid onto the patient’s anatomy. This real-time Cherenkov visualization enables immediate verification of beam delivery and surface dose distribution, enhancing treatment accuracy and patient safety.
By leveraging Cherenkov imaging, this approach provides a non-invasive method for monitoring radiation dose deposition in real-time, improving the accuracy of treatment verification and potentially enhancing patient safety in radiotherapy.
Photobiomodulation (PBM)
Photobiomodulation (PBM) is a non-invasive therapy that uses low-level red or near-infrared light to stimulate cellular processes, promoting tissue repair, reducing inflammation, and alleviating pain. The therapeutic use of light dates back to ancient civilizations, where sunlight was believed to have healing properties. Today, PBM is widely applied in medicine, dentistry, and rehabilitation for wound healing, neuroprotection, and muscle recovery. Our research in PBM focuses on PBM dosimetry, aiming to optimize light parameters such as wavelength, fluence rate, and exposure time to achieve precise therapeutic effects while ensuring safety and efficacy.