Archived Featured Faculty: Theresa Busch
Theresa Busch, Ph.D.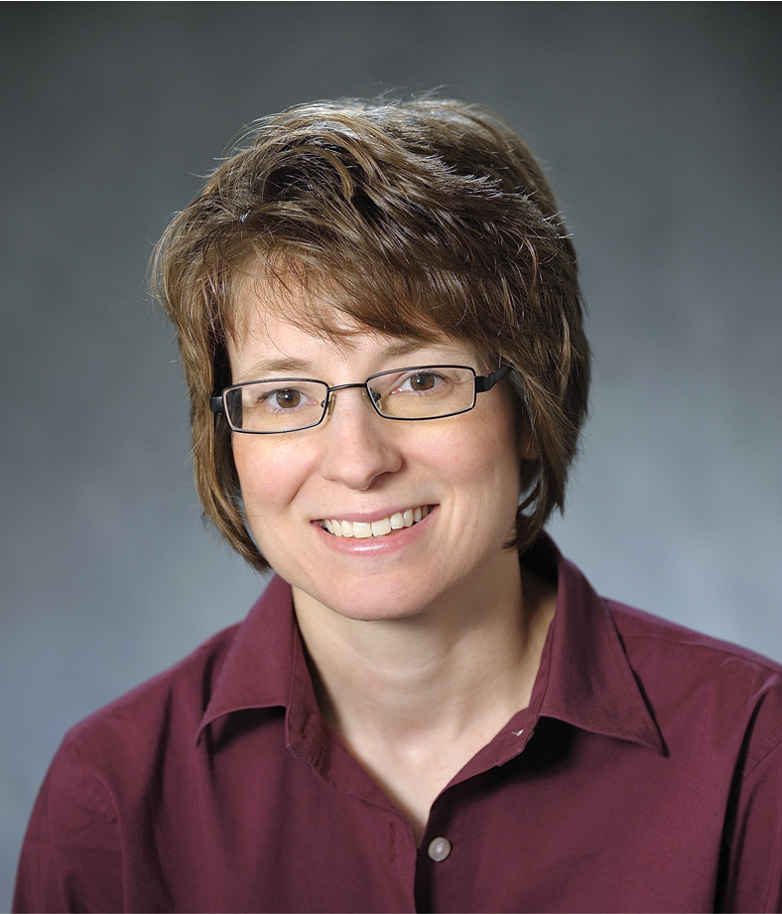
Vice Chair for Inclusion, Diversity and Equity
Associate Director of Research and Research Professor
Department of Radiation Oncology
University of Pennsylvania
Telephone: 215-573-3168
Email: theresa.busch@pennmedicine.upenn.edu
Dr. Theresa Busch received her PhD in Biophysics from the State University of New York at Buffalo, Roswell Park Cancer Institute Division, and completed a postdoctoral fellowship at the University of Pennsylvania. Presently, Dr. Busch is Vice Chair for Inclusion, Diversity and Equity in the Department of Radiation Oncology, Associate Director of Radiation Oncology Research and Research Professor. Dr. Busch’s laboratory performs translational research in the study of tumor microenvironment as it relates to radiation therapy, delivered as nonionizing radiation in the form of photodynamic therapy or ionizing radiation generated by photons or protons. Her laboratory has interrogated both the dependencies and consequences of radiotherapy on microenvironmental factors such as tumor hypoxia, inflammation, and blood vessel composition and function. Using mechanistic knowledge that is gained from these studies, her laboratory investigates clinically relevant approaches to improve the therapeutic window of cancer treatment.
Dr. Busch’s research has been continuously supported by the NIH since 2001 through her leadership of R21, R01 and P01 grants. These grants provide resources for an interdisciplinary research program that comprehensively supports scientific inquiry across the spectrum of bench-top through clinical trial research. In addition to her involvement with the Center for Targeted Therapeutics & Translational Nanomedicine, Dr. Busch is a member of the Radiobiology and Imaging Program of the Abramson Cancer Center, faculty of the Biochemistry and Molecular Biophysics Graduate Group, and scientist appointee to the Institutional Animal Care and Use Committee. She is a standing member of NIH study section and on the Executive Council of the American Society for Photobiology.
Several areas of research being pursued by the Busch lab are highlighted below:
The biological impact of dose rate in radiotherapy. A long-standing interest in the Busch laboratory has been the study of how outcomes to photodynamic therapy (PDT) are determined by the effective dose rate, altered by changing the radiation (light) fluence rate. More recently, we are also investigating the parallel concept of absorbed dose rate as an effector of outcomes to proton therapy. These radiotherapy parameters are determined by the treatment protocol, for example FLASH versus standard dose rate in proton therapy or high versus low fluence rate in PDT. Moreover, at a chosen treatment prescription, inhomogeneity in dose rate exists in tissue. In preclinical studies, we have identified that differentials in PDT fluence rate will distinctively impact tumor microenvironment, leading us to consider the distribution of fluence rates across the thoracic cavity during a clinical trial of PDT of malignant pleural mesothelioma (MPM). In PDT of MPM, the illumination source is systematically moved throughout the surgically-exposed chest, and in this way provides comprehensive treatment that is monitored via optical detectors at eight locations in the chest cavity. We observed fluence rate to be quite consistent across these eight tissue sites for measurements averaged across patients (Figure 1a). Conversely, when fluence rates were reported by patient (averaged across the eight sites within each patient), certain patients emerged as experiencing higher or lower treatment-averaged fluence rate, yet importantly, all patients received moderate fluence rate overall (Figure 1b). Accompanying measurements of tissue oxygenation (tissue hemoglobin oxygen saturation, StO2) revealed that patients with higher pre-PDT tissue oxygenation experienced a decrease in oxygenation after treatment (Figure 1c), suggesting that better oxygenation prior to PDT promoted physiological changes consistent with tissue damage.
Figure 1. (a) Treatment-averaged fluence rate at each of eight tissue sites in patients receiving PDT for MPM. Box plots of data at each tissue site include measurements averaged over 15 patients. Tissue sites include: apex, anterior chest wall (ACW), posterior chest wall (PCW), posterior mediastinum (PM), diaphragm (Diaph), pericardium (Peri), posterior sulcus (PS), and anterior sulcus (AS). (b) Treatment-averaged fluence rate in each of 15 patients receiving PDT for MPM. Box plots of data for each patient include measurements averaged over 8 tissue sites at which light was measured. Red indicates patients exposed to intermediate fluence rate and green those exposed to low fluence rate. (c) Tissue hemoglobin oxygen saturation (StO2) plotted for tissue sites with a StO2 ratio<1 (meaning oxygenation decreased after PDT; 40 tissues) or a StO2 ratio>1 (meaning that oxygenation increased during PDT, 26 tissues). Tissues with higher StO2 before PDT were more likely to experience PDT-induced decreases in oxygenation, as would be consistent with treatment-introduced damage.
References:
- Diffenderfer ES, et al. Design, Implementation, and in Vivo Validation of a Novel Proton FLASH Radiation Therapy System. Int J Radiat Oncol Biol Phys. 2020;106(2):440-448. PMID: 31928642
- Dupre PJ, et al. Light Fluence Rate and Tissue Oxygenation (StO2) Distributions Within the Thoracic Cavity of Patients Receiving Intraoperative Photodynamic Therapy for Malignant Pleural Mesothelioma. Photochem Photobiol. 2020 Mar;96(2):417-425. PMID: 32048732
Clinical studies of lesion oxygenation. The significance of tissue oxygenation has been further explored in other clinical trials of PDT, demonstrating a relationship between the pre-existing oxygenation of lesions and treatment response. For example, in a clinical trial of PDT for premalignant/early stage head and neck cancer, we evaluated the oxygenation (StO2) of oral lesions (Figure 2a, b), comparing these data to clinical outcome (Figure 2c). Based on these measurements, it was found that patients whose intact lesions were more highly oxygenated prior to PDT were more likely to achieve 24-month complete response.
Figure 2. (a) Schematic of the system for diffuse reflectance/fluorescence spectroscopy that provides clinical assessment of tissue oxygenation (tissue hemoglobin oxygen saturation, StO2) and tissue levels of the photosensitizing drug for PDT that sensitizes cells to specific wavelength of visible light. On the probe face, the reflectance (R) and fluorescence (F) sources, as well as the first 4 detection fibers (A through D) are labelled. (b) Photographs of the handheld probe and its face. (c) Kaplan Meier plots of PDT response in patients with intact lesions of the head and neck, segregated by high (n=12) versus low (n=13) baseline oxygenation and showing high oxygenation (StO2) pre-PDT to associate with 24-month complete response (p=0.02).
References:
- Gallagher-Colombo SM, et al. Measuring the physiologic properties of oral lesions receiving fractionated photodynamic therapy. Photochem Photobiol. 2015;91(5):1210-8. PMCID: PMC4560604
- Ahn PH, et al. Lesion oxygenation associates with clinical outcomes in premalignant and early stage head and neck tumors treated on a phase 1 trial of photodynamic therapy. Photodiagnosis Photodyn Ther. 2018;21:28-35. PMCID: PMC5866751
Personalizing treatment via tumor hemodynamics. In parallel with our interests in tumor oxygenation and hemodynamics, we have investigated how light delivery for PDT can be modulated in order to control treatment-introduced physiologic changes in tumors. Our goal is to develop a platform for real time adjustment of light delivery for PDT optimization (Figure 3a). In animal models, we have established that changes in tumor oxygenation and blood flow during light delivery are predictive of therapeutic outcome. In these models, we have employed interactive light delivery that is guided by real-time physiologic measurements (Figure 3b) in order to optimize treatment effect (Figure 3c).
References:
- Yu G, et al. Noninvasive monitoring of murine tumor blood flow during and after photodynamic therapy provides early assessment of therapeutic efficacy. Clin Cancer Res. 2005;11(9):3543-52. PMID: 15867258
- Ong YH, et al. Blood flow measurements enable optimization of light delivery for personalized photodynamic therapy. Cancers. 2020 Jun 15;12(6):E1584. PMID: 32549354
Augmenting radiotherapy through innate immunity. Another focus of our research lies in defining and augmenting innate immune responses to PDT. We have studied structural and molecular factors that determine how tumor blood vessels react to treatment. This incorporates work that assesses the role of inflammation and neutrophils in therapeutic response, including in the context of multimodality therapy. The broader significance of our findings is derived from the support they lend to strategies for introducing vascular “priming” or immunotherapy drugs to augment innate immune response to radiotherapy. Recent studies have considered how the extent of neutrophil activation by photodynamic therapy associates with treatment outcome (Figure 4).
Figure 4. Luminol imaging demonstrating higher PDT-induced signal (reporting neutrophil activation) in mice that go on to achieve a durable complete response (90 days) to PDT of their tumor. Images depict baseline (pre-PDT) and 1h post-PDT luminol signal at the treatment site in representative mice, separated as a function of treatment outcome. Box plots summarize results from all animals. Filled circle in recurrence group indicates outlier. N = 5 for Complete Response and N = 15 for Recurrence. *P = 0.025 for the comparison between mice with a complete response versus recurrence.
References:
- Davis RW 4th, et al. A preclinical model to investigate the role of surgically-induced inflammation in tumor responses to intraoperative photodynamic therapy. Lasers Surg Med. 2018;50(5):440-450. PMID: 29799130.
- Davis RW 4th, et al. Luminol chemiluminescence reports photodynamic therapy-generated neutrophil activity In vivo and serves as a biomarker of therapeutic efficacy. Photochem Photobiol. 2019; 95(1):430-438. PMCID: PMC6472895.
- Cramer GM, et al. Photodynamic Therapy and Immune Checkpoint Blockade. Photochem Photobiol. 2020 Jun 23. Online ahead of print. PMID: 32573787