Research in the Arany Lab
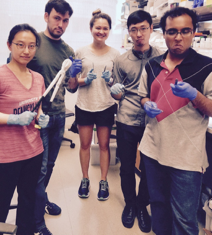
The Arany lab is interested in all things cardiovascular metabolism. The ideal project leverages multi-disciplinary tools and approaches, and spans from molecular mechanistic work, to murine models of disease, to human studies. We take multidisciplinary approaches, ranging from molecular biology and high-throughput metabolomics (e.g. C13 flux analyses) and genomics to cell biology, mouse physiology, and human genetics. Our goal is to understand events that underlie physiological and pathological metabolic adaptations in heart, skeletal muscle, and the vasculature.
Current Topics of Interest
Understanding cardiac metabolism is at the core of the lab’s interests.
We have published extensively on this topic for 15 years, including seminal work on the role of PGC-1a in the heart. More recently, we demonstrated a surprise role for adenine nucleotide transporter (ANT) in controlling mitophagy, with important implications for cardiac disease (Nature 2019). We are currently highly focused on human cardiac metabolism (see this review: Cell Metabolism 2024). For example, we worked with Penn Medicine Electrophysiologists and LC-MS metabolomics evaluation of arterio-venous changes in plasma nutrients, using blood from artery and coronary sinus, to provide a comprehensive map of human cardiac fuel consumption (Science 2020). And we worked with the Margulies lab to expose changes in cardiac metabolism in human failing cardiac tissue (Nature Cardiovascular Research 2022). We then leverage these observations in humans to carry out mechanistic studies in mice, such as our recent deep dive into the role of branched chain amino acids in heart failure (Cell Metabolism 2022). Most recently, we have identified novel metabolic targets of the widely used “SGLT2 inhibitors”, most notably pantothenate kinase (PANK), which may explain how these inhibitors have such profound cardiometabolic benefits independently of SGLT2 itself (Circ Res 2024, BioRxiv 2024). Current projects vary widely, including: ongoing human A/CS studies and other approaches to learn about cardiac metabolism from human subjects; generation and evaluation of genetic mouse models of metabolism in the heart; a deep dive into PANK biology; and studies focused on right heart failure, an often ignored but clinically significant component of heart failure.
(1) How is vascular metabolism regulated by the underlying parenchyma (e.g. skeletal muscle or heart)? We published seminal work identifying PGC-1a in skeletal muscle as a key driver of cross-talk with endothelial cells, driving angiogenesis (Nature 2008) and transport of nutrients (Nature Medicine 2016). Projects are ongoing to further understand the molecular mechanisms of endothelial-parenchymal crosstalk, with a particular focus on fatty acid transport (Cell Metabolism 2020) and its consequences on insulin resistance and diabetes. (2) How does metabolism within the endothelial cell affect vascular function? Endothelial cells are metabolically fascinating: largely quiescent, but metabolically very similar to tumor cells, including having a strong Warburg effect at baseline. We have recently reported on surprising roles of glycolytic enzymes (JCI 2018), glutamine enzymes (EMBO 2017), NAD-consuming SIRT1 (Cell 2018), lactate (EMBO 2022), acetate (Cell metabolism 2023), and most recently lipid droplets (JCI 2024) in endothelium. Numerous active projects in the lab include understanding the role of NAD biology and lipid handling in the endothelium, and the consequences on diabetes, heart failure, and atherosclerosis.
Branched chain amino acids (BCAAs: leucine, valine, and isoleucine) have taken center stage recently as potential contributors to insulin resistance, heart failure, and other pathologies.
We reported that 3-hydroxyisobutyrate, a metabolite of valine, acts as a paracrine signal to promote lipotoxicity and insulin resistance in muscle (Nature Medicine 2016). This led us to a comprehensive study of how BCAAs are handled, partitioned, and oxidized by the entire organism, using state-of-the-art LC/MS-based studies on live conscious mice infused at steady-state with heavy isotope tracers (Cell Metabolism 2019), and subsequently to the generation of various mouse alleles in BCAA catabolic enzymes to probe in depth the role of BCAA catabolism in muscle, liver, and heart, in insulin resistance and heart failure (Cell Metabolism 2022, and Nature Metabolism 2023). Most recently, we have leveraged these novel techniques to comprehensively quantify metabolic fluxes in mice during acute cold stress (Cell Metabolism 2023) and during acute exercise (Cell Metabolism 2024). Active projects include understanding molecular mechanisms of regulation of BCAA catabolism, and testing the role of BCAA catabolism in endothelium, pancreatic cancer, and renal cancer. We are also extending our in vivo steady state isotopic approaches to understand systemic fluxes of nutrients during heart failure and in cardiac/kidney metabolic disease (CKM).
Getting at the heart of pregnancy and peripartum cardiomyopathy (PPCM).
~1:1000 women in late pregnancy or recently pregnant mysteriously develop profound heart failure, known as peripartum cardiomyopathy (PPCM), often leaving them incapacitated at a critical moment in their life and that of their child. We have made two seminal advances to understanding this disease: first, that PPCM is in part driven by anti-vascular hormones secreted by the placenta, i.e. PPCM is a vasculo/hormonal disease (Nature 2012). And second, that ~10% of women with PPCM bear loss-of-function mutations in the gene TTN, encoding for the large sarcomeric protein titin (NEJM 2016). A major focus of the lab currently is to probe more deeply into the genetics of PPCM, using international cohorts (Circulation 2021); to understand racial disparities PPCM (JAMA Cardiology 2017); medRxiv 2024); and to understand how TTN mutations cause disease (Circulation 2019; Science Trans Med 2021; J Clin Invest 2024), with the ultimate goal to identify treatments for this devastating disease. As part of this effort, we also recently established a patient-directed North American Peripartum Cardiomyopathy Registry (PPCM-R), the first of its kind. We also recently reviewed the state of the field (NEJM 2024). Current efforts include trying to understand how hormones emanating from the placenta reprogram maternal cardiovascular physiology and contribute to disease. This work is in part supported by a Leducq Consortium on the role of the placenta in maternal and fetal cardiovascular health.
The mTOR pathway is central to cellular metabolic regulation, but it is not monolithic.
Surprisingly, until our recent work, the mTORC1 complex was largely described as a single switch that translates dozens of inputs into dozens of outputs. But no one has only one light switch in their house. Over the past few years, we have championed the notion that mTOR is instead a switchboard, in which some upstream signals affect only some of the downstream readouts. We have clearly delineated the first such example, involving the specific regulation by mTOR of the TFE family of transcription factors through a FLCN-mTORC1-TFE axis. We have worked out molecular mechanisms (PLoS Biology 2021) and evaluated its implication in adipose tissue (Genes and Development 2016), monocytes (JCI insight 2019), and most recently hepatocytes, demonstrating a dramatic protection from MAFLD/MASH by modulation of this pathway (Science 2022). Active projects include understanding the impact of modulating this pathway on lipid handling in the liver including VLDL secretion, its impact on hepatocellular cancer, understanding the feedback loops of this complex pathway, and exploring translational possibilities in the context of MAFLD/MASH.
COVID-19 has devastated the world. Cardiovascular disease, including cardiomyopathy, are a prominent feature.
Preexisting cardiovascular and metabolic diseases are strong risk factors for adverse clinical course, and cardiac damage is one of the strongest predictors for rates of fatality. We have ongoing projects evaluating the effects on SARS-CoV2 on vasculature and cardiomyocytes, testing the hypothesis that direct cardiovascular damage to these cells by the virus underlies adverse clinical outcomes.
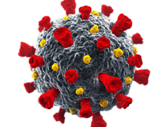