Research
The strength and fixation of orthopaedic implant designs presents a variety of biomechanical and clinical challenges. By blending mechanical, computational, and basic science techniques, we continue to explore the clinical problems associated with orthopaedic implant form and function. Our work has focused on thoroughly investigating and challenging the existing paradigms used to fixate bone, in an effort to reduce the incidence of malunion, minimize the time required before return to activity, and improve the overall standard of care. Specifically, we are interested in optimizing the stiffness of repair constructs by isolating and testing design elements that may directly influence stabilization. We have found that current implant designs may be overly rigid for the elderly population, and we are currently seeking new ways to tailor implant designs to adapt to pathologic bone stock.
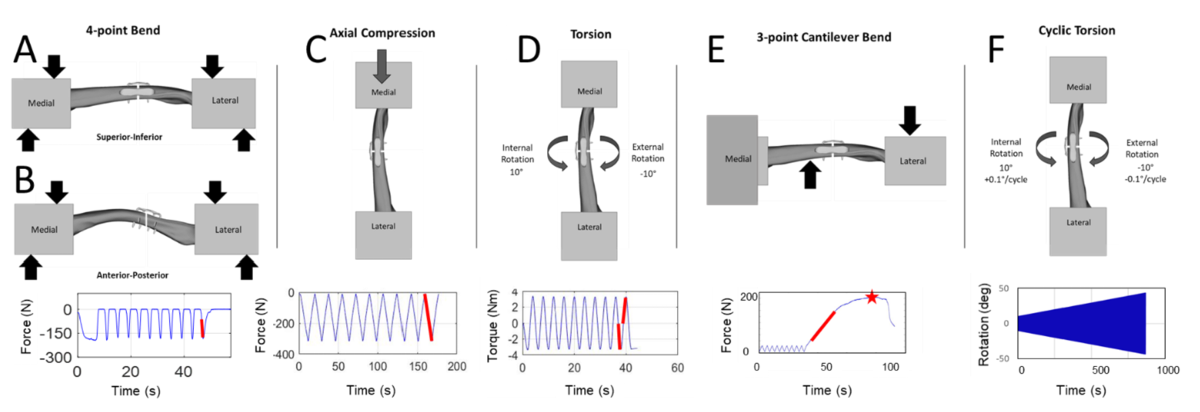
Selected Publications:
- Schmidt EC, Dear KA, Hendow C, Miller L, Mehta S, Hast MW. (2021) Examining the novel use of continuous compression implants in clavicle reconstruction: A biomechanical study. Clin Biomech (Bristol, Avon). Aug;88:105437. PMID: 34311318.
- Hast MW, Chin M, Schmidt EC, Sanville J, Van Osten GK, Mehta S. (2020) Mechanical Effects of Bone Substitute and Far-Cortical Locking Techniques in 2-Part Proximal Humerus Fracture Reconstruction: A Cadaveric Study. J Orthop Trauma34(4):199-205. PMID: 32197036.
- Mehta S, Chin M, Sanville J, Namdari S, Hast MW. (2018) Calcar screw position in proximal humerus fracture fixation: Don't miss high! Injury. 49(3):624-629. PMID: 29452734; PMCID: PMC7413303.
- Mehta S, Chin M, Sanville J, Namdari S, Hast MW. (2018) Use of an Additional Nonlocking Screw in Olecranon Fracture Osteosynthesis Changes Failure Mechanism. Orthopedics. 42(1):e74-e80. PMID: 30484851; PMCID: PMC7410497.
Additive manufacturing (AM) processes are changing the way the world thinks about the design and manufacture of physical objects. Orthopaedic implants are no exception. Although this technology is still in its nascency, there are plenty of clinical applications for 3-D printed implants. Our work in this area has explored the biomechanics of AM implants in comparison to those made with traditional subtractive processes. We have found that AM implants not only provide similar mechanical strength and fixation, which allows for the creation of a variety of design goals. Examples include patient-specific design geometries, introduction of porous surfaces to improve bone ingrowth, and adaptation of mechanical strength without changing exterior geometry. Based on the work we have done in this area, we believe additive manufacturing has tremendous potential to substantially shift paradigms of clinical care in a very short period of time.
Selected Publications:
- Tilton M, Lewis GS, Hast MW, Fox E, Manogharan G. Additively manufactured patient-specific prosthesis for tumor reconstruction: Design, process, and properties. PLoS One. 2021 Jul 14;16(7):e0253786. doi: 10.1371/journal.pone.0253786. PMID: 34260623; PMCID: PMC8279401.
- Inacio JV, Cristino DM, Hast MW, Dailey HL. An Adaptable Computed Tomography- Derived Three-Dimensional-Printed Alignment Fixture Minimizes Errors in Radius Biomechanical Testing. J Biomech Eng. 2021 Nov 1;143(11):111006. doi: 10.1115/1.4051433. PMID: 34114605.
- Tilton M, Armstrong AD, Wee HB, Hast MW, Manogharan G, Lewis GS. (2020) Finite Element-Predicted Effects of Screw Configuration in Proximal Humerus Fracture Fixation. Journal of biomechanical engineering. 142(8):081005.
- Tilton M, Lewis GS, Wee HB, Hast MW, Manogharan G. (2020) Additive manufacturing of fracture fixation implants: Design, material characterization, biomechanical modeling and experimentation. Additive manufacturing. 33:101137.
- Tilton M, Armstrong A, Sanville J, Chin M, Hast MW, Lewis GS, Manogharan GP. (2020) Biomechanical Testing of Additive Manufactured Proximal Humerus Fracture Fixation Plates. Ann Biomed Eng. 48(1):463-476. PubMed PMID: 31555983
The role of computational simulations in orthopaedics continues to gain importance and utility as we progress through the second decade of the 21st century. These simulations can provide insight into mechanisms within orthopaedics, such as joint loads, which are impossible to measure in vivo. Our work has been dedicated to the development and use of musculoskeletal models to predict performance characteristics of orthopaedic implants. These projects have proven to be capable of quickly and accurately predicting the kinematics and kinetics associated with activities of daily living in patients that have had surgical reconstruction of a joint or bone. By providing a non-invasive, cheap, and quick method to predict patient-specific implant performance, this research has added to the critical mass that improves the speed and accuracy in which implant performance can be fully characterized and better understood. Additionally, this approach can has improved efficiency of the ever-evolving design cycle of implants.

Selected Publications:
- Hast MW, Piazza SJ. (2013) Dual-joint modeling for estimation of total knee replacement contact forces during locomotion. J Biomech Eng. 135(2):021013. PubMed PMID: 23445058.
- Hast MW, Hanson BG, Baxter JR. (2019) Simulating contact using the elastic foundation algorithm in OpenSim. J Biomech. 82:392-396. PubMed PMID: 30501910.
- Hast MW, Schmidt EC, Kelly JD 4th, Baxter JR. (2018) Computational optimization of graft tension in simulated superior capsule reconstructions. J Orthop Res. 36(10):2789-2796. PubMed PMID: 29761555.
- Hast MW, Piazza SJ. (2018) Position of the quadriceps actuator influences knee loads during simulated squat testing. J Biomech. 73:227-232. PubMed PMID: 29576314.
Tendons, ligaments, and soft tissue all play an important role in the efficient transfer of forces from muscle onto the skeletal frame of the body. To date, the fundamental mechanisms that drive tendon growth, maintenance, and repair are still not clearly understood. We conduct experiments that explore the fundamental aspects tendon and ligament structure, function, injury, healing, repair, and regeneration. This basic understanding of soft tissue biomechanics provides valuable information to develop and evaluate potential treatment modalities. These research questions are typically investigated with cadaveric or animal models, which permits the investigation of basic science questions that remain unanswered about such tissues, and open the door to future clinical trials.

Selected Publications:
- Freedman BR, Kuttler A, Beckmann N, Nam S, Kent D, Schuleit M, Ramazani F, Accart N, Rock A, Li J, Kurz M, Fisch A, Ullrich T, Hast MW, Tinguely Y, Weber E, Mooney DJ. (2022) Enhanced tendon healing by a tough hydrogel with an adhesive side and high drug-loading capacity. Nat Biomed Eng. doi: 10.1038/s41551-021-00810-0. Epub ahead of print. PMID: 34980903.
- Schmidt EC, Chin M, Aoyama JT, Ganley TJ, Shea KG, Hast MW. (2019) Mechanical and Microstructural Properties of Pediatric Anterior Cruciate Ligaments and Autograft Tendons Used for Reconstruction. Orthop J Sports Med. 7(1):2325967118821667. PubMed Central PMCID: PMC6348523.
- Zelenski NA, Schmidt E, Chin M, Gittings D, Steinberg D, Hast MW. (2021) Flexor Pollicis Longus Tendon Wear Associated With Volar Plating: A Cadaveric Study. J Hand Surg Am. 46(2):106-113. PubMed PMID: 32950317.
- Baxter JR, Farber DC, Hast MW. (2019) Plantarflexor fiber and tendon slack length are strong determinants of simulated single-leg heel raise height. J Biomech. 27;86:27-33. PubMed PMID: 30722935.