Our Research
Our lab has a long-term interest in understanding the fundamental aspects of skeletal muscle and cardiac function in normal or diseased conditions and in the practical aspects of manipulating these functions by using animal models and tissue engineering approaches for treatment intervention.
We believe that combining bioengineering approaches with stem cell biology, testing cell-based therapies and modulating the signaling pathways of adult stem cells we can enhance the replacement of skeletal muscle and cardiac tissue upon injury. We are presently combining genetic knockout mice and gene recombination with cellular engineering techniques to investigate the temporal and spatial communication of cells and signaling pathways during muscle regeneration.
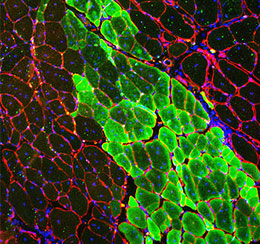
Disruption of skeletal muscle integrity results in muscle atrophy and degeneration but the cellular and molecular underpinnings that will promote regeneration remain elusive. We showed that inhibition of NF-κB pathway induces regeneration after injury and protects against denervation-induced muscle atrophy (JCI, 2016). Furthermore, our research revealed a muscle stem cell-autonomous increase in the activity of the p38 mitogen-activated protein kinase pathway (Nature Medicine, 2014) and provided the first evidence that polarization of macrophages (from pro-inflammatory to anti-inflammatory) is fundamental for skeletal muscle regeneration in mice (PNAs, 2009). Previously, we developed a new mouse model (dystrophic mice with shorten telomeres, mdx/mTR mouse) that better replicates the phenotype of Duchenne Muscular Dystrophy (DMD), providing novel evidence that the skeletal muscle phenotype is caused by a failure of skeletal muscle stem cells to maintain the damage-repair cycle initiated by dystrophin deficiency (Cell, 2010).
Current Research
Our current research has 3 connected thematic areas:
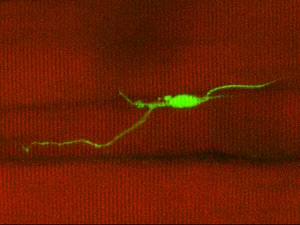
Mechanobiology and morphological heterogeneity in muscle stem cells. A first research focus is understanding the role of MuSCs under injury. For this purpose, we generated a new mouse model (Pax7EGFP), where EGFP expression is both robust and dynamic and a real-time indicator of MuSC stemness (Skeletal Muscle, 2018). This genetically modified model can be used to study essential questions related to MuSC biology, by itself or used in combination with mice harboring additional genetic alterations. Importantly, we found that this mouse line has allowed us to reliably monitor the dynamic behavior of MuSCs in live animals (Star Protocols, 2023), the first time this has been possible in the field. We demonstrated that adult quiescent MuSCs are categorized into functional distinct subtypes and discovered that the mechanosensing signaling pathway Piezo1 regulates their properties during regeneration. Contrary to earlier thinking that quiescence is a passive and dormant state lacking activities, our work reveals that cellular quiescence in skeletal muscles is actively maintained and that it corresponds to a collection of morphological heterogeneous states (Science Advances, 2022). Such breakthrough studies provide us with the unique ability to address fundamental questions into the emerging cell biology of stem cells.
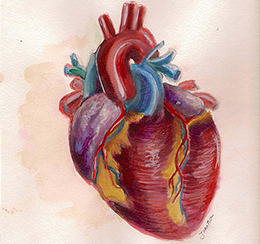
Telomere biology in cardiac and skeletal muscles. The second research theme is on Duchenne Muscular Dystrophy (DMD), the most common inherited form of myopathy, affecting both skeletal and cardiac muscles. Repeated cycles of muscle damage and repair in DMD lead to stem cell dysfunction in skeletal muscles. Using a powerful and innovative single-cell imaging technique we developed to monitor diseased adult muscle stem cells (MuSCs), we discovered that telomere shortening is a key feature of dystrophic MuSCs in both mice and DMD patients, starting at a very young age (Stem Cell Reports, 2018). Importantly, we discovered the underlying mechanism behind such molecular alterations. We demonstrated for the first time that prolonged NF-κB activation in diseased mouse MuSCs leads to shortened telomeres, independent of cell replication, via a mechanism that involves Ku80 alterations and excessive DNA damage to telomeres. As a result, there is premature telomere uncapping in diseased MuSCs leading to stem cell exhaustion (Cell Reports, 2021). Since the major cause of death in DMD is cardiac failure, we extended our studies to heart tissue and measured telomere length in a cell-type specific manner that bypasses previous limitations by avoiding contributions of undesired cell types in cardiac tissues (Nature Protocols, 2017). We discovered an important mechanistic role for telomeres in human cardiomyopathy and demonstrated shortened telomeres only in cardiomyocytes of DMD patients. Furthermore, we provided the first evidence of a direct association between cardiomyocyte-specific telomere shortening and human heart failure, highlighting its significance in aging and gender and demonstrated that telomere shortening is a previously unrecognized signature of cardiac diseases (J Am Heart Assoc, 2017).
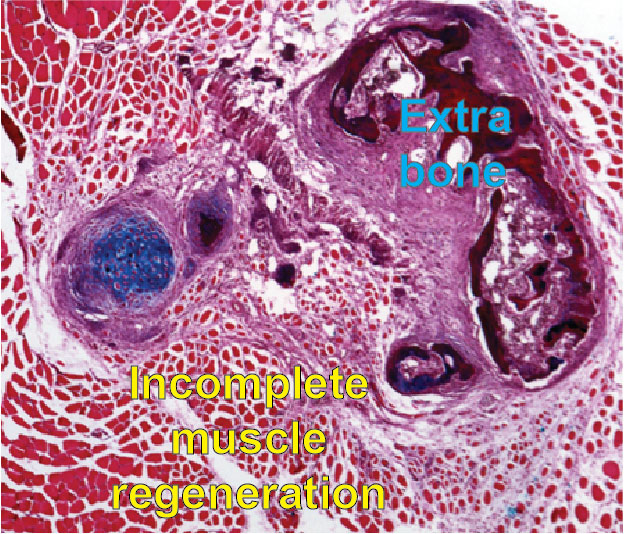
and heterotopic bone formation
Communication of muscle progenitor cells (MuSCs-FAPs) during regeneration. In a third and related research project, we investigated the dynamics of two adult resident stem cells (MuSCs and Fibro-adipogenic progenitors, also called FAPs) during skeletal muscle regeneration. We identified for the first time that Gli1 (a crucial mediator of Hh signaling), labels a distinct subset of FAPs. We discovered that in the absence of Gli+FAPs, skeletal muscle repairs poorly with abnormal intermuscular accumulation of fat (JBMR, 2021). We have extended these studies to address a major challenge of muscle regeneration in a rare disease called Fibrodysplasia ossificans progressiva (FOP), in which upon injury, heterotopic (extra-skeletal) bone forms within skeletal muscle. Initially, we showed that elevated BMP signaling alters cellular mechanotransduction and muscle microenvironment after injury (J Bone Miner Res, 2019). More recently, we demonstrated that FOP MuSCs fail to proceed normally during myogenesis, due to an improper communication with FAPs during muscle regeneration. These data reveal a fundamental role of FAPs in influencing the myogenic activity of MuSCs in this rare disease after injury and, as a consequence, MuSC-FAP coordination may be an important target for future therapeutic interventions to improve the health of those with FOP (NPJ Regen Med, 2021, mdpi Biomedicines, 2024).